Nitrogen New Routes
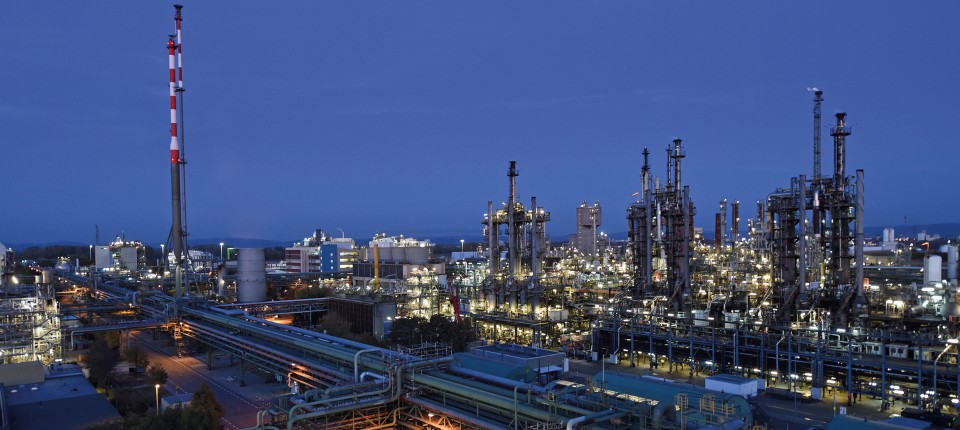
Ammonia is a cornerstone of our agriculture. However, the current methods of synthesis are anything but optimal. This could change soon. What some microorganisms have managed to do seemingly effortlessly for billions of years, humans can only do with great effort: Humanity invests more than one percent of global energy production in the synthesis of nitrogen compounds.
The starting material is atmospheric nitrogen, which is abundant. About 78% of the Earth’s atmosphere consists of this gas. The product of so-called nitrogen fixation is usually ammonia (NH3). In particular, synthetic fertilizers are produced from it, without which there would be no agriculture capable of feeding eight billion people.
Fritz Haber and Karl Bosch, who later won the Nobel Prize, successfully fixed the first technical nitrogen in 1913. Since then, ammonia has been produced from hydrogen (H2) and nitrogen using the Haber-Bosch process. For the reaction to take place, in addition to the appropriate catalysts, high temperatures and pressures of around 500 °C and 200 bar are required – and therefore a lot of energy. Atmospheric nitrogen exists as “N2”, i.e. molecules composed of two nitrogen atoms each.
To form other nitrogen compounds, the bond between the two atoms in the N2 molecule must be broken. However, this is one of the strongest chemical bonds of all. The microorganisms mentioned at the beginning now have evolutionarily improved biological catalysts called nitrogenases to fix nitrogen in the air and thus make it usable for all other organisms in the food chain. Shouldn’t human chemistry also be able to obtain nitrogen compounds more efficiently?
One way to make industrial nitrogen fixation more energy efficient is to facilitate the separation of ammonia from the Haber-Bosch reactor. Until now, it had to be cryoliquefied and then distilled. Heating and cooling consumes large amounts of energy.
A new development from a California research group led by Jeffrey R. Long may help here: Scientists report a new material in the journal Nature that efficiently binds ammonia, stores it, and releases it again when needed. It’s called a MOF (for metal-organic framework), a metal-organic framework through which gases can move freely.
Copper atoms, which like to bond and incorporate ammonia molecules into the surrounding structures of the structure, are located at certain points in the metallic structure. On the other hand, other gases leave the pores undisturbed. The ammonia can later be expelled again by the use of vacuum and heating, thus replenishing the storage material. As the researchers report, their MOFs can be used to remove ammonia directly from the manufacturing process — no distillation, no liquefaction, and therefore no refrigeration.
Store, Link, Edit
Other research efforts are aimed at finding new ways to chemically capture nitrogen. Until a few years ago, metals in particular were examined here for their suitability as catalysts – that is, as substances that participate in a chemical reaction, potentiating or enabling it in the first place, but without being consumed in the process.
But in the meantime, scientists have opened up large portions of the periodic table of chemical elements to fix nitrogen: even elements like the light, non-metallic boron are able to react with nitrogen in the atmosphere – and even allow for previously unknown interactions, such as bonding direct flow of two N2 molecules. However, the most promising processes still use minerals. The long-term goal of all this research is to develop new catalysts that can operate at lower temperatures or use alternative energy sources such as electricity or light.
Furthermore, the catalyst should be as inexpensive as possible, available in large quantities, non-toxic and chemically robust. Computer-aided simulations are now making an important contribution to catalyst research. As a kind of virtual laboratory, they can help to screen a large number of candidates in a short period of time. In this way, the number of laboratory experiments can be greatly reduced, which saves costs and protects the environment at the same time. Furthermore, computer operations allow deep insights into the functioning of catalysts, thus supporting their targeted development.
The Energy has to come from somewhere.
Japanese chemists working with Yoshiaki Nishibayashi provide an example of photocatalysis, that is, light-mediated nitrogen fixation in Nature Communications. Their process uses two catalysts: one made of iridium that captures visible light and boosts the other made of molybdenum, which now handles the actual nitrogen fixation.
A special feature of this process is that, instead of elemental hydrogen, an organic molecule called dihydroacRidin is used as a type of hydrogen carrier that uses tz to convert nitrogen into ammonia. Unfortunately, dihydrocridine is not suitable for large scale synthesis and large amounts of an unusable by-product are obtained.
However, the work shows that such a process could, in principle, also work without hydrogen and without high temperatures. On the other hand, other works focus on improving process control. A group at the Max Planck Society for Coal Research in Mülheim developed a Ruhr der process that produced ammonia even at room temperature and normal pressure.
They use a ball mill as a reactor, where the mechanical friction of the balls drives the chemical reaction. However, what works in the laboratory cannot always be individually implemented in industry, and the path from promising development to full-scale application is often a long one. Even here. It wasn’t until the 1990’s that a modified process, which used a ruthenium catalyst instead of Fritz Haber’s original iron catalyst, was put into operation.